What happens when you sprain your ankle? Does the ligament rupture, or is it stretched? How long does it take before it heals? Does it fully recover?
One might think that the answers to these questions are common knowledge for doctors and physiotherapists, but they are not. The questions seem simple enough, but in reality we cannot give a definite answer to any of them, but here we will try anyway. In the following text, I will sometimes refer to tendons and sometimes to ligaments, although most of what is written applies to both. Tendons can be different, as can ligaments, but in broad terms we can consider them the same, as long as we exclude the finger flexor tendons, which are a special case.
With regard to the first question, how can we find out whether the ligament ruptures or is stretched on injury? It is possible to try and reason out what the answer is, but then we need to know the structure of a ligament, what it can endure and how elastic it is, that is how much it can be stretched and then spring back without being damaged.
Tendons are strong; it would be possible to suspend a small car from an Achilles tendon. We can think of tendons and ligaments as bundles of fine filaments lying in a parallel arrangement and embedded in a sticky, jelly-like substance. The threads are, of course, the collagen fibres and the other material is mostly proteoglycans, large molecules with a certain amount of absorbent capacity. The collagen fibres are long and could possibly stretch along the whole length of the tendon without any joins. There are cells sparsely scattered here and there, and their function is to watch over the tendon material and replace it when necessary. This is a slow process, and how it works is not really known. When the tendon is not under traction, the collagen fibres lie in elongated spirals. This is seen as a wave-like pattern under the microscope (called crimp-pattern) and is caused by elastin fibres contracting the tendon.
The main function of a tendon is to pull. To investigate how it carries out this task, a tendon can be mounted in a tensile testing machine. The machine distends the tendon at a constant speed, and an inbuilt meter records the force that the tendon resists. The results are usually plotted on a curve where distension is shown on the x-axis and force on the y-axis. At the beginning of the tensile test, the resistance is weak as only the elastin fibres are stretched. When the collagen spirals are pulled out so that the fibres are straight, the force increases. For every millimetre that the tendon is stretched the force increases by the same amount, so the curve is a steeply rising straight line. During distension, mechanical energy is supplied to the tendon. If the machine is stopped and reversed back to the starting point, nearly all the energy is returned. The tendon acts like a spring and only a small amount of energy is lost; around 15%, as heat loss. This provides the background to a normal, yet remarkable, human behaviour, namely that of being able to stand and jump on the spot, at a rave party or whilst country dancing. The spring-like properties of the tendons allow us to keep doing this all night without being too impeded by loss of energy. They are also essential, for example, for long distance running and high jump.
Tensile test of a tendon or ligament
However, back to the tensile testing machine. What happens if the tendon continues to be pulled? When stretching to somewhere between 5% and 8%, small dents in the curve will be seen before it levels off and suddenly drops to zero. The tendon has ruptured. The small dents appear because individual fibre bundles have snapped. This can happen anywhere, so that small micro-injuries occur in many different places along the tendon. This means that when the tendon ruptures completely, due to these small tears connecting with each other, the injury then becomes frayed like a broken rope. If we manage to stop the machine after the curve has started to level off, but before the tendon ruptures completely, we would get a structure that is permanently elongated by a few percent. I believe that such injuries are fairly uncommon. To return to the sprained foot; in general this is either dispersed micro-injuries or a total rupture. Any significant distension will hardly ever occur. However, many tendons and ligaments consist of different parts that are subject to different loadings. One part may rupture whereas another holds. This is what we call partial ruptures.
Without access to a tensile testing machine, one can of course cut open the skin of the injured person and take a look. This was not unusual during the 1970s, particularly in athletes that fell into the hands of enthusiastic sports physicians who were of the opinion that all injuries should be stitched. The ligaments in question had almost always completely ruptured, but of course only the most pronounced injuries were cut open. Nowadays, injuries can be examined using ultrasound, where most of the slightly more severe ankle sprains are seen to probably involve at least one completely ruptured ligament.
When a tendon has ruptured, how does it heal?
First of all, the healing process needs to start; but how do the cells know that there has been an injury and that a healing response is required? There are thought to be two possible mechanisms. The first is bleeding. When the ligament ruptures then its blood vessels are also damaged. Platelets in the blood become activated when they suddenly come into contact with proteins not normally found in the bloodstream. They release the growth factors they contain, which can trigger a number of the surrounding cells. The other mechanism depends on molecules from the interior of the cells that ruptured from the injury. being released. When they are seen by the immune system, they are perceived as foreign and dangerous. This leads to inflammation, and a number of inflammatory cells are attracted to the site.
The inflammation is initially non-specific and not much different from the reaction to invading bacteria, but for unknown reasons, the process changes in character and the inflammatory cells, in particular the macrophages, switch from combating imaginary enemies to stimulating reconstruction. They summon endothelial cells that can form new blood vessels and mesenchymal stem cells that can start forming a new ground substance with collagen. At first, the new collagen is sparse and of lower quality (type lll collagen). This new material looks like a watery gel; a pink coloured granulation tissue, which is also seen after some time in open skin wounds and called proud flesh. The collagen is haphazardly organised, but the new tissue is plentiful. It becomes a large nodule (tendon callus) that completely encloses the old injury site. The cells obviously prioritise quantity rather than quality. However, the callus is reasonably strong, partly due to its size. The tissue has elastic properties right from the start; if pulled out and then released it will immediately regain its shape. This is important in the next phase in the development: remodelling and functional adaptation.
For the new tissue to gain more tendon-like properties, the collagen needs to achieve a higher quality and, above all, be organised in neat bundles aligned in the direction of strain. The cells can in fact secrete collagen in such a way that the fibres end up in the desired orientation, but how do they know which direction is correct? The cells cannot detect force. “Force” is in fact an abstract concept that is difficult to comprehend and it is only because we are so accustomed to the idea of forces that we are unaware of this. However, cells can detect changes in shape. If the muscle pulls the damaged tendon and then releases it then we have a deformation cycle that the cells are able to detect. They also then receive the information they require regarding orientation. This is why the elasticity of the early callus is important; the tissue must be able to be pulled out and then return to its original shape in order to receive information about orientation.
The cells now successively break down the early callus and replace it with a better, more functionally adapted material, primarily type I collagen, now more tightly packed and in the correct orientation. The callus decreases in size and finally we end up with something that resembles the original tendon. The joins with the former tendon stumps have also disappeared.
How similar is the new tissue to an undamaged tendon? This probably varies. It is unclear whether the new tissue is best described as a mature scar or a new tendon; the boundaries here are diffuse. There are athletes who have regained top function in their ruptured Achilles tendons, but in general I believe that primarily a part of the energy storage capacity of the tendon is not fully restored. In animal experiments on ligaments and tendons, small islands of fatty tissue are sometimes seen in the new material. This fat has been thought to come from neighbouring fatty tissue that has ended up in the wrong place during injury, which has been an argument for surgical treatment of injuries. Personally, I believe that there is evidence to suggest that the fat comes from stem cells that have been involved in the reconstruction process, but have misunderstood their task. Mesenchymal stem cells have a strong tendency to form fat cells if they do not receive other directives. These fatty islands may act as shock absorbers and therefore impair the energy saving properties, at the same time as the tissue becomes a little less stiff. On the other hand, scar tissue is often remarkably stiff.
The healing process is usually described as having three phases. The fact that the number of phases is three has nothing to do with biology, but rather with the poetic triad. In any case, the three phases are inflammation, regeneration and remodelling. Pain occurs during inflammation even when at rest, during regeneration under normal load bearing, and during remodelling with extreme loading. Inflammation lasts a week, regeneration a month and remodelling a year. It goes without saying that these are gross generalisations, but easy to remember (thanks to the triad!).
Is it possible to influence the healing process?
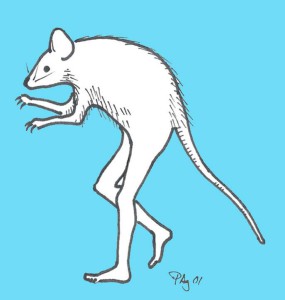
The ideal laboratory animal for research on Achilles tendons
To answer this question we are almost exclusively referred to research studies on animals. My research group has been carrying out such studies for 20 years, and we still know far too little. In order to study a biological process in a model, it is necessary to simplify and standardise, as well as keep the costs down. We mostly use rats, where we quite simply cut the Achilles tendon and stitch up the skin although not the tendon. A defect of several millimetres develops which fills with blood. After a few days, granulation tissue can be seen, after about a week the rat can walk “on its toes” again and after three weeks the tendon is as strong as before (although by no means completely remodelled).
Healing can be stimulated in rats by injecting substances, but this is hardly potential for development for human use, unfortunately.
We succeeded early on in accelerating the healing process with a single injection of a growth stimulating protein (GDF 5, 6 or 7) in the hematoma, but we never succeeded in getting the pharmaceutical industry interested in this. We therefore tested platelet-rich plasma (PRP) instead. Since platelets contain many growth factors and are thought to trigger the healing process, we thought that more of a good thing could be even better. It worked extremely well! We were able to increase the tensile strength by 50% after a week, using just a single injection, and confirmed this in several test series in our rat model. Encouraged by this, we carried out a randomised clinical trial in patients with ruptured Achilles tendons. This showed no beneficial effect whatsoever, and possibly some deterioration. That’s the way it goes sometimes! It is difficult to apply the results from an animal model to humans. There are many conceivable explanations for the differences, although hardly any of them mean that we had missed a beneficial effect for patients. Unfortunately, our experiments on rats have prompted people in many parts of the world to treat various sports injuries with injections of platelet-rich plasma without conducting randomised trials. It remains to be seen if the negative results from studies in humans will get them stop.
Can conventional medicines influence the healing of tendons?
Yes indeed! First and foremost cyclooxygenase inhibitors (NSAIDs: Ibuprofen, Diclofenac, Celecoxib etc) have a clear inhibitory effect on tendon healing in our rat model. A normal dose for the first five days is enough to reduce strength by a third after a week. It takes a long time before it catches up with the untreated control tendons. Cyclooxygenase inhibitors are, of course, anti-inflammatory so these findings demonstrate that the early inflammation is necessary to give the healing process a good start. Even if we only have data from rats, it is likely in this case that the effects are the same in humans. Cyclooxygenase inhibitors have the same effect on the healing of fractures, and we know that this effect is similar in both rats and humans. There is a large randomised study that shows a great increase in the percentage of complications during healing in patients with high-energy fractures of long bones who are given NSAIDs. Yet, we often use NSAIDs to give pain relief with fractures. This is not ignorance, but a calculated risk. Common low energy fractures almost always heal well, and it may be worth a possible delay in healing so that good pain relief can be given without using morphine preparations. However, fractures where there is a risk of healing problems should definitely not be treated with NSAIDs.
Back to tendons and ligaments. If I were to sprain my foot I would take NSAIDs, even though they delay healing, just to reduce the pain and be able to start walking on my foot as soon as possible. On the other hand, I believe that the bad practice of treating top athletes almost continuously with NSAIDs definitely increases the risk of long-term problems.
What does mechanical loading mean for healing?
I have already described how mechanical loading is necessary to give the cells information about how the collagen should be oriented. This is what can generally be found in textbooks. However, loading is much more important than that. If we remove mechanical loading from our rat model, then only a thin, immature tissue is formed with a strength of 15-20% of what is otherwise normally seen. If we only moderately reduce the possibility for the rat to load the tendon, we get perhaps 30% of the strength found in the control animals. The callus is similar in size to the completely unloaded tendons, but the material properties are similar to the control tendons. This means that only a small amount of loading is required to get the right material properties, but more loading is required in order to get a thick callus.
Development of stiffness over time after rupture of the human Achilles tendon. The healing process in human Achilles tendons seems to prioritise quantity over quality. The stiffness of the newly formed materials does not increase at all from week 7 to week 19 after injury (patients do not exercise until week 7). At one year, the material properties have finally improved.
In general it is recommended that injuries should be allowed “to rest” during the inflammatory phase, and that mobilisation should begin at the earliest when the inflammation has subsided and the regenerative phase has started (many probably do not dare to start then either). We have tested this “clinical truth” in our rat model and found, completely unexpectedly, that loading during the first 6 days had an equally favourable effect as loading during the regenerative phase. It is obvious that loading in some way favourably modifies inflammation.
We also investigated the length of time required for loading to produce a beneficial effect and concluded that 5 minutes once a day is enough. If the time is extended to 15 minutes then a better effect is obtained but increasing the time any further has little benefit. We have some evidence that resting between these short episodes of loading is favourable so that, amongst other things, the tissue is able to shorten.
Use the force!
Loading triggers a complex cellular machinery, which is no doubt different in different cells, and many of the hundreds of genes that are activated are to do with inflammation. For instance, the synthesis pathway of PGE2 is stimulated. This is the substance that lies behind all the symptoms that the use of NSAIDs tries to relieve, from the common cold to menstrual pain and pain from a foot sprain. It is maybe here that the explanation for the adverse effects of NSAIDs on healing lies. If PGE2 is required for the beneficial effect of loading, then NSAIDs would counteract that effect. However, this remains to be demonstrated.
We have investigated which genes are activated first after a single loading. It was found to be only four genes, all general activators that can trigger all kinds of things. What happens later and what guides the process towards tendon healing must therefore depend on diverse environmental factors. Although, this is only partly true; two of these general activators also have, among many other functions, the specific task of triggering the formation of tendon tissue during fetal development!
We have, of course, considered whether loading also stimulates healing in our patients. Many probably think this is obvious, but it is not. This problem has been studied with regard to ruptured Achilles tendons. It was found that patients given a hinged brace or who are allowed to take off the brace and move their foot now and again are more satisfied and feel that the tendon performs better. However, this does not necessarily mean that it has increased in strength. The treatment effect may be a result of a reduction in oedema around the ankle and the avoidance of other side effects of immobilisation. Furthermore, it is possible to wiggle the foot and also walk on it, without subjecting the Achilles tendon to traction.
We therefore carried out a study where patients with a ruptured Achilles tendon received surgery (it is not at all certain that this is better than no surgery) and then were randomised into groups, where one group used a stiff brace for 7 weeks and the other group a brace that after 3 weeks could be removed twice a day. The patient was then allowed to load the Achilles tendon 20 times using a simple apparatus that increased the resistance every week.
During surgery, the patients had received small metal balls inserted into the tendon. By allowing patients to load the tendon at the same time as it was x-rayed, we could measure how the distance between the metal balls increased with a known load and thereby calculate the stiffness of the tendon. The group that was allowed to load the tendon was found to have a substantially stiffer tendon both after three months and one year! However, they did not notice any difference themselves.
Patients who were allowed to pedal (loading) had greater stiffness after 19 and 52 weeks.
Further reading?
This account is based mainly on research carried out by my own research group. Confirmation of nearly everything that has been stated above can be found by searching in PubMed for “Aspenberg P AND tendon”. A number of people have carried out this research, including Carina Forsberg, Olena Virchenko, Pernilla Eliasson, Therese Andersson, Malin Hammerman, Anna-Carin Lundin and Thorsten Schepull. The summaries and reference lists in Olena’s, Pernilla’s and Thorsten’s theses from Linköping University can give a good overview.